Engineers
are often prone to making mistakes. That is why we constantly have to
check and double-check everything that we do. It is this type of keen
focus on what we do that allowed generations of past engineers to create
some of the most remarkable engineering projects.
However, details are sometimes overlooked, numbers misrepresented or
even units misread. While some of these mistakes are miniscule and can
be corrected, history has witnessed some colossal oversights that led to
huge disasters and in some cases, popular travel destinations.
The Leaning Tower of Pisa
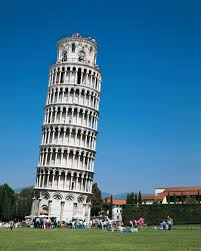
One of the world’s greatest attractions is actually a result of an
engineering error. For over 800 years, the leaning tower of Pisa
continues to draw worldwide attention and is a popular destination for
tourists. Construction began in 1173 on an unstable foundation that
comprised of mud, sand and clay. When engineers got to the third floor,
the tower began to sink into the soft soil and lean on one side. They
tried to fix the problem by making the columns and arches of the third
story on the sinking northern side slightly taller. However,
construction halted due to political unrest and only resumed a century
later. The tower was closed in 1990 for safety reasons and millions of
dollars were poured in to stabilize the structure and set it back to the
position it had in 1838. Engineers added cables to stabilize the
structure, then excavated under the tower and added trusses and
counterweights.
Tacoma Narrows Bridge
Known as the “Galloping Gertie”, the original Tacoma Narrows Bridge
was opened in July 1940 and at the time it was the third longest
suspension bridge in the world. Its nickname was derived from the
bridge’s sensitivity to high winds, causing it to sway and vibrate. Just
four months after opening, the structure collapsed though its only
fatality was a black Cocker Spaniel. Engineers failed to account for the
aerodynamic forces within the location, especially during periods of
strong winds. Thus the bridge was vulnerable to vibrations generated by
wind. A replacement bridge was constructed ten years later, after the
end of the Second World War. The remains of the original bridge remain
at the bottom of Puget Sound, where they form one of the largest
man-made reefs in the world.
Chernobyl Nuclear Power Plant
On 26 April 1986, a structurally unsound reactor in the Chernobyl
Nuclear Power Plant, located in Ukraine, exploded. It was the worst
nuclear power plant disaster in history, resulting in a severe nuclear
meltdown. Highly radioactive materials were discharged into the
atmosphere and over an extensive geographical region following the
explosion. It spread to as far as Italy and to date over 500,000 deaths
have been linked to this catastrophe. By May, about 116,000 people that
had been living within a 30-kilometre radius had been evacuated and
later relocated to safer regions. The accident was caused by a flawed
reactor design that was operated with inadequately trained personnel and
poor safety regulations.
New Orleans Canal and Levee System
In 2005 Hurricane Katrina hit New Orleans, devastating the city and
flooding about 80 percent of the region, killing thousands and
displacing several families. The U.S. Army Corps of Engineers were found
liable for this devastation which was compounded by an antiquated levee
and canal system that protected the city. The levees failed because
they were built in a disjointed fashion, were inconsistent in quality,
materials and design and outdated data was used that left gaps exploited
by the storm. Additionally, engineers did not take into account the
poor soil quality underneath New Orleans. Since then the U.S. government
has spent more than $15 billion to upgrade the system.
Deepwater Horizon Spill
One of the worst environmental disasters in U.S. history, the
Deepwater Horizon occurred in April 2010 after an explosion tore through
a British Petroleum drilling rig. 11 crew members were killed and it is
estimated that 180 million gallons of oil was released into the gulf.
Over 8,000 animals were reported dead just 6 months after the spill and
16,000 total miles of coastline were affected, including the coasts of
Texas, Louisiana, Mississippi, Alabama, and Florida. Mechanical failure
as well as human error led to this colossal catastrophe. The engineers
repeatedly ignored the well’s orneriness and chose to take quicker,
cheaper and ultimately more dangerous actions that eventually led to the
total well blowout.
Space Shuttle Challenger
Just a few seconds after the space shuttle challenger was launched in
January 1986, it broke apart and killed everyone aboard. It resulted
due to the failure of the solid rocket booster O-rings to seal properly,
allowing hot combustion gases to leak from the side of the booster and
burn through the external fuel tank. Though the problems with the
O-rings had been known for nine years, engineers continued to ignore it
as they assumed safety was ensured with the presence of the second ring.
Eager to launch the shuttle, NASA managers also ignored warnings from
engineers that low temperatures could exacerbate the problem.
Banqiao Reservoir Dam
Built in the early 1950s as party of a huge project to control
flooding and produce electricity in central China, the Banqiao Reservoir
Dam could hold back almost 500 million cubic meters of water. A
hydrologist called Chen Xing warned that overbuilding of dams (over 100
were built in that period) and reservoirs could raise the water table in
Henan beyond safe levels and lead to disaster. Furthermore, the dam was
only built with 5 sluice gates when Xing warned that it needed at least
12. In August 1975, Typhoon Nina dropped more than a year’s worth of
rain in just 24 hours and the dam failed. It released the equivalent of
280,000 Olympic-sized swimming pools, taking with it entire towns and
killing as many as 171,000.
The Boston Molasses Disaster
Towering over Boston’s North End, construction ended on a massive
molasses tank that stood 50 feet tall, 90 feet in diameter, and held
more than 2 million gallons of molasses. The tank would help sate the
USA’s appetite for industrial alcohol, largely for use in the munitions
business. However, in their haste to make a profit, the owners
overlooked the wisdom in hiring skilled engineers and instead sought out
a man who was unable to read blueprints or even order a simple stress
test. As a result, the tank exploded without warning and caused a wave
of molasses and debris to travel down the street at 35 miles per hour.
At 25 feet high, it ripped buildings off their foundations, killed 21
people and injured 150 others.